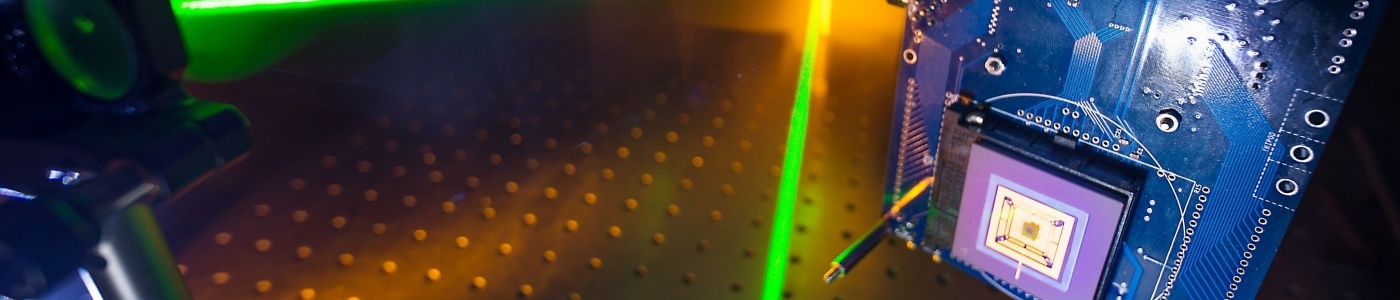
Quantum sensors and imaging can aid the defence industry to see through fog and smoke and assist with covert operations
Sensing and Imaging for Defence and Security
At QuantIC we are developing a range of cameras and sensors that will advance surveillance, navigation, and threat detection across defence and security.
Quantum effects allow us to surpass classical limits to reduce image noise or enhance image resolution - enabling covert surveillance from greater distances, allowing visibility through fog, smoke or dust and round corners.
Quantum imaging can also provide an alternative approach to navigation informing position location without reference to satellites.
Quantum Imaging for Defence and Security
Discover how researchers at the University of Southampton are making the infrared visible.
Quantic technology for defence and security
REAL TIME 3D IMAGING
Most conventional cameras only see in 2D. By using real-time technology, we can time how long it takes light to bounce back from the object, producing a 3D image. This is prime technology for depth imaging through complex and challenging conditions such as fog, smoke, turbid water or when camouflaged by foliage.
Building upon the work in QuantIC phase one, we are using pulsed illumination and SPAD sensor arrays to record the range of each image pixel (>10,000 pixels) simultaneously. Results have shown centimetre depth resolution at 10s or even 100s metre range and frame rates up to 30Hz. The sensor data is denoised using sophisticated data processing to give 3D representations of the surrounding environment.
UNDERWATER IMAGING
Imaging through clear and clean water can be quite straightforward. However, once the water becomes turbid, the high degree of back-scattered light saturates the detector and the image can no longer be recognised. One approach to overcoming this backscatter is to use short-pulse illumination and time-resolved single-photon detection so that the returned photons can be seen above the back-scattered background level.
We are using both SPAD detector arrays and single-detector, raster scanned systems to suppress backscatter from the recorded images. In both cases the timing resolution corresponds to a few millimetres in depth and by rejecting all the other photons we have shown imaging at over 9 scattering lengths (measured one way) at ranges of several metres.
Such systems have significant application within sub-sea operations, for example in offshore installation inspection and terrain mapping and hence navigation. We are presently building a demonstration unit for trials in real-world conditions.
WAVELENGTH TRANSFORMATION CAMERA
Conventional infra-red cameras use esoteric semiconductors and detect the light directly but with a much lower sensitivity and slower speed than is possible in the near-visible region of the spectrum using cameras based on silicon. Infra-red cameras based on these other semiconductors are also vastly more expensive than silicon cameras, largely because the volumes produced are much lower, but also are typically noisy because of the smaller bandgap of the semiconductor compared to silicon.
At QuantIC, we are using non-linear optical materials to convert the longer infrared light into near-visible light so that it can then be imaged using conventional, cheap, high-performance cameras based upon silicon technology. However, up until now no one has managed to do this with both high efficiency and in a multi-pixel configuration.
Contributions that we are making to the field include, putting the non-linear material in a waveguide configuration to increase the conversion efficiency and using multiple waveguides and scanning systems in various configurations to make a multi-pixel device. The waveguide configuration can reach a conversion efficiency for infra-red to visible light of 40%. In achieving this goal we will bring visible detector performance to infrared imaging systems.
Possible applications vary from eye-safe (>1.5μm) imaging with visible detectors for automotive LIDAR.
NON-LINE OF SIGHT IMAGING
Traditional imaging relies upon direct line of sight between object and camera. However, point a laser at the ground in front of you and light scatters in all directions - around corners or perhaps it hits an out-of-view object.
We are using both SPAD detector arrays and single detector systems to detect the single photons of this scattered light. Each scattering point creates a circle of light, much like the ripple that is created by dropping a stone in a pond. By observing these ripples, we can locate the object and work out both its size and speed even though it lies out with the line of sight. Our results so far show that we can detect objects 2-3m around a corner.
SINGLE-FIBRE ENDOSCOPE
Traditional endoscopes are based on bundles of many 1000s of optical fibres, one fibre for every pixel in the image. A less intrusive endoscope could be based on a single-fibre the width of a human hair. However, when an image is transmitted along a single-fibre, cross-talk between modes, scrambles the light such that the transmitted image is unrecognisable.
However, using advanced computing, beam shaping, and photon timing allows a 3D image to be extracted from this noise, making an endoscope the width of a human hair possible. Such systems are potentially of relevance to both security and surveillance applications.
IMAGING WITH UNDETECTED PHOTONS
Central to all conventional imaging is the notion that the light which illuminates the object is the light that is detected by the image sensor. However, in quantum science this light can be linked (entangled) to light of a different wavelength.
The image encoded on infra-red light used to illuminate an object can, after transmission through the object, be transferred to visible light.
QuantIC are using new structured materials to generate entangled photons further into the infra-red, targeting the wavelengths used to detect hydrocarbon gases.
If such systems are realised, then it becomes possible using standard cameras to image at many wavelengths across the electromagnetic spectrum, potentially reaching as far as the terahertz regime for application in covert detection and screening.
CASE STUDY: Kromek radiation detectors
International technology group Kromek partnered with QuantIC researchers at the University of Glasgow to investigate the possibility of developing 3D imaging of gamma radiation.
They were attracted by the University’s research and development of Timepix chips - integrated circuits that are suitable to combine with multiple different detectors developed by Professor Val O’Shea.
Kromek worked in collaboration with QuantIC researchers to investigate the feasibility of integrating CdZnTe with Timepix 3 and through the development of new imaging algorithms obtain suitable imaging performance that can be exploited within a new range of gamma ray imaging instrumentation.
The project was successful and Kromek licensed aspects of the Timepix technology, software and readout electronics developed at University of Glasgow. This was applied by Kromek to enable 3D reconstruction of gamma ray environments and they have rolled out gamma ray detectors to the medical, civil nuclear and security sectors.